Virus and Bacteria Deactivation Research with Energetiq Tunable Light Sources
Author: Bill Grube
The COVID-19 pandemic has impacted the lives of millions worldwide. As of August 31, 2020, there have been over 25 million cases confirmed. The number of deaths related to COVID-19 exceeds 800,000 and continues to rise. In terms of mortality, the COVID-19 virus does not act alone. In fact, early evidence suggests that approximately 50% of the people who have passed from COVID-19 also had a secondary infection.1 These secondary infections are known as superinfections and are a complication in which an additional infection occurs on top of the primary viral infection. Superinfections contribute to the overall mortality rate associated with COVID-19, as patients are exposed to bacteria and viruses that lead to superinfections in public spaces and healthcare facilities.
Key Advantages of Energetiq's TLS
- Broad wavelength ranges
- High resolution (narrow bandwidth)
- High in-band light flux
- High spatial and long term stability
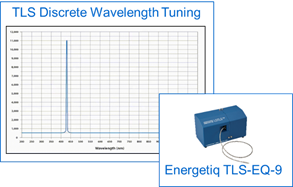
Different wavelengths of light have been demonstrated to deactivate specific bacteria and viruses. Researchers are working to identify the wavelengths that are effective against various pathogens and are also safe for human exposure. The COVID-19 pandemic has motivated increased research in this area. Energetiq’s Tunable Light Sources (TLS) are ideal tools for this research. Each TLS model is built around a Laser-Driven Light Source (LDLS™) with a customized monochromator platform. The high spectral radiance of the LDLS enables the combination of high spectral resolution and high light throughput that is needed for this important work.
Light-Based Virus and Bacteria Deactivation
Historical Context - Therapeutic Benefits of Light
The earliest record of sunlight as a medical treatment dates back to the time of the Egyptian Pharaohs in 5000 BCE.2 Egyptians treated chronic ulcers with exposure to the sun, and sun-based health practices have been documented in ancient Babylonia, Mesopotamia, Greece, and Rome.
Far-UV Wavelengths
The ability of UVC light (200 nm-280 nm) to deactivate pathogens has long been established. However, UVC light presents its own health risks because it can contribute to an increased risk of skin cancer and damage to the eyes.3, 4 Recent research demonstrates that Far-UVC light (207 nn – 222 nm) is effective in deactivating bacteria and is safe for the skin and eyes.5-7
Blue Wavelengths
Evidence shows that violet/blue light (400 nm – 470 nm) is antimicrobial against numerous bacteria, and that it accounts for Niels Ryberg Finsen's Nobel-winning treatment of tuberculosis.2 Further evidence shows that blue light deactivates several viruses, including the common flu coronavirus.8 Atmospheric ozone absorbs much of the ultraviolet (UV) content of sunlight before reaching the surface of the earth. In fact, the amount of blue and violet light reaching the earth is ten times more than the amount of UV. Given the absorption of blue/violet light by most microbes and the resulting bactericidal effect, it is reasonable to attribute a good proportion of the sun's environmental sanitization to blue/violet wavelengths.2, 9
Red Wavelengths
In experimental animals, red and near-infrared (NIR) light has been shown to reduce respiratory disorders, similar to complications associated with coronavirus infection.² In patients, red light has been shown to alleviate chronic obstructive lung disease and bronchial asthma.²
Energetiq’s family of Tunable Light Sources
Operating principles
The Energetiq Tunable Light Source (TLS) pairs Energetiq’s unique Laser-Driven Light Source (LDLS™) with a customized monochromator that is matched to the characteristics of the source to maximize coupling efficiency. This combination offers both the high resolution and high light throughput needed for virus and bacteria deactivation research.
The monochromator of the TLS scans light by wavelength. The width of the entrance and exit slits of the monochromator plays a key role in the tradeoff between wavelength bandwidth and in-band light flux. Wider slits allow more light to pass, but with a broader bandwidth. Conversely, narrower slits enable a narrow bandwidth (high resolution), but also restrict the amount of light that can be delivered. The TLS is unique because of the high spectral radiance of the LDLS around which the product is built. The high radiance of the LDLS source allows more light to be efficiently coupled through a narrow monochromator slit. This means that more light flux can be delivered within a narrow bandwidth than is possible with other tunable light sources. The amount of light delivered through the Energetiq TLS monochromator slit, as compared to that of a typical xenon arc lamp-based system, is illustrated in the two inset diagrams of Figure 1. The spectral radiance of the LDLS is approximately 7-times higher than that of a xenon arc lamp, enabling higher light throughput.
TLS models and performance
Energetiq Tunable Light Sources are available in several models, optimized for different wavelength ranges and output levels. Below is a summary of the options that are currently available.
Part Number | Description | Wavelength Range (nm) |
---|---|---|
TLS-EQ-9
|
Vis/NIR standard
|
380 - 1100 (nm)
|
TLS-EQ-77
|
Vis/NIR higher power
|
350 - 1100 (nm)
|
TLS-EQ-77-UV
|
200 - 770
|
Label Text
|
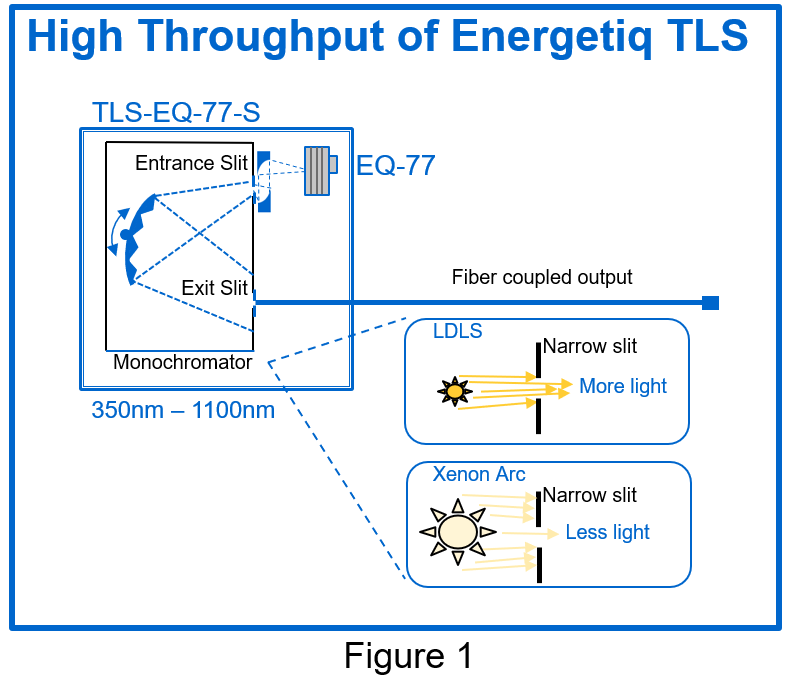
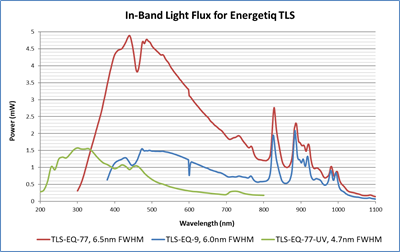
Conclusion
Energetiq’s family of tunable light sources is comprised of flexible tools that provide unique benefits for research in the area of wavelength-specific light deactivation of common bacteria and viruses, including COVID-19.
References
- Michael J. Cox, Nicholas Loman, Justin O’Grady, Co-infections: potentially lethal and unexplored in COVID-19, The Lancet Microbe,, April 24, 2020.
- Chukuka Samuel Enwemeke, Violet Vakunseh Bumah, Daniela Santos Masson-Meyers, Light as a potential treatment for pandemic coronavirus infections: A perspective, Journal of Photochemistry & Photobiology, B: Biology, 27 April 2020.
- Setlow, R. B., Grist, E., Thompson, K. & Woodhead, A. D. Wavelengths effective in induction of malignant melanoma. Proc Natl Acad Sci USA 90, 6666–6670 (1993).
- Balasubramanian, D. Ultraviolet radiation and cataract. J Ocul Pharmacol Ther 16, 285–297 (2000).
- Buonanno, M. et al. 207-nm UV light - a promising tool for safe low-cost reduction of surgical site infections. I: in vitro studies. PLoS One 8, e76968 (2013).
- Buonanno, M. et al. 207-nm UV Light-A Promising Tool for Safe Low-Cost Reduction of Surgical Site Infections. II: In-Vivo Safety Studies. PLoS One 11, e0138418 (2016).
- Buonanno, M. et al. Germicidal Efficacy and Mammalian Skin Safety of 222-nm UV Light. Radiat. Res. 187, 483–491 (2017).
- C.S. Enwemeka, Antimicrobial blue light: an emerging alternative to antibiotics, Photomed. Laser Surg. 31 (2013) 509–511.
- P.E. Hockberger, The discovery of the damaging effect of sunlight on bacteria, J.
- Photochem. Photobiol. B 58 (2000) 185–191.
Updated: August 2021