LDLS™ Powered Tunable Light Source with Far-UVC Output
Author: X. Ye, M. Alfaris, W. Grube, D. Gustafson, H. Zhu
Abstract
Tunable light source powered by LDLSTM with far-UVC output is proposed and evaluated. Experimental results of in-band fluxes, FWHM bandwidths between 200 nm and 400 nm, are presented.
OCIS codes: (120.0120) Instrumentation, measurement, and metrology; (120.4820) Optical systems
Introduction
The pandemic from the recent outbreak of COVID-19 calls for rapid mobilization of every possible clinical tool, including phototherapy, which has been demonstrated to be one of the most effective ways to reduce the impact of the 1918 “Spanish influenza” pandemic [1]. Evidences show that far-UVC light (207 nm–222 nm) efficiently inactivates bacteria without harm to exposed mammalian skin, since far-UVC light cannot penetrate even the outer layers of human skin or eye due to its strong absorbance in biological materials [2]. Further evidence shows that UV and blue light can inactivate several viruses, including the common flu coronavirus [3]. These findings attract more attention and efforts to further explore the clinical value of light, which is helpful to avoid another pandemic to serve as a reminder. The unique property of wavelength tunable light sources, especially with capability of tuning range covering from far-UVC to visible, makes it suitable to function as a key role in the research and development in phototherapy activities, as researchers can manipulate desired wavelength to observe the response from the virus or bacteria under test.
In this study, a TLS powered by an LDLSTM with specific design to facilitate UV output is presented. The output wavelength could cover from visible to UVC as deep as 200 nm. The light emitting Xe plasma in the LDLS is sustained by focusing a high power laser beam into the Xe plasma [4]. LDLS sources are superior than other light sources including higher spatial and temporal stability, smaller plasma size, higher brightness, longer lifetime, and lower maintenance. The FWHM for the output could be configured to desired value by adjusting the slits width in the monochromator.
Schematic and Principles
A schematic of the LDLS powered TLS with high UV output is shown in Fig.1. The operation principles of an LDLS could be reached in an earlier publication [5]. In design, an LDLS (model EQ-77) serves as the light source for the LDTLS. A spherical retroreflector with enhanced UV coating is aligned to image the Xe plasma back onto itself for a higher forward emitting radiance. Achromatic reflective optics including two off-axis-parabolic (OAP) mirrors (3, 4) with UV reflectance coating are optimized to etendue-match the LDLS and the monochromator (6) to maximize optical throughput. OAP-3 has 2" effective-focal-length (EFL) for light collection from the LDLS, and beam collimation as well; OAP-4 has an 8" EFL, functioning as beam focusing to the UV monochromator. The UV monochromator is a diffraction grating based wavelength selector which functions dissolving and selecting single wavelength to the output. The width adjustable slit is designed for wavelength bandwidth control for the output signal to facilitate different requirements. The output from the UV monochromator is coupled into a 600 µm core diameter UV fiber for easier beam deployment in applications.
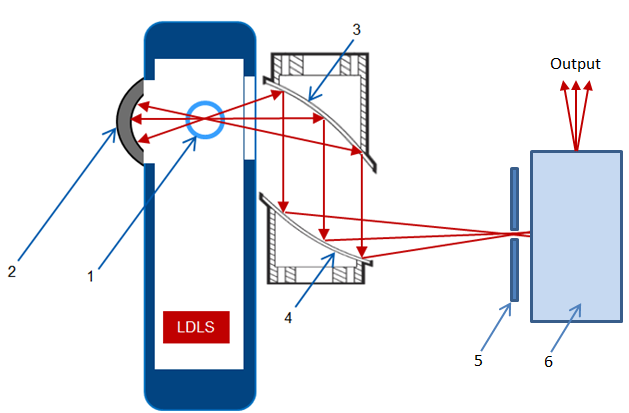
1- High-pressure Xe bulb
2- Retroreflector
3- OAP mirror
4- OAP mirror
5- Width adjustable slit
6- UV monochromator
Fig.1: Schematic diagram for LDLS based UV tunable light sources
Measurement Results & Analysis
Figure 2 shows the output performance of the TLS, where (a) is the in-band fluxes from 200 nm to 400 nm, (b) shows spectral density for the TLS. As seen in Figure 2 (a), the in-band flux range is between 329 µW (at 200 nm) and 1574 µW (at 300 nm), with a flux average of 1171.7 µW. The spectral density shown in Fig. 2 (b) ranges from 284.5 µW/(nm*mm2) to 1124.6µW/(nm*mm2). The min spectral density locates at 210 nm and the max locates at 300 nm. The spectral density average is 819.2 µW/(nm*mm2, which is much higher than current broad-band UV light sources on market.
Fig.2 In-band flux and spectral density for the UV LDTLS, (a) in-band fluxes from 200nm to 400nm; (b) spectral density for the TLS
Figure 3 presents spectral performance for the TLS, with (a) showing the FWHM bandwidth from 200 nm to 400 nm, and (b) showing spectral output at 260 nm, and (c) showing spectral output when the wavelength set to 400 nm. The FWHM bandwidth ranges from 3.4nm to 6.2nm with an increasing trend versus wavelength. The mean value of the FWHM is 5.1nm. The spectral output at different wavelength is consistent with slight FWHM variation, as shown in Fig.3 (b) and (c).
Fig.3 Spectral performance for the UV LDTLS, (a) FWHM bandwidth over 200 nm to 400 nm range; (b) spectral output at 260 nm; (c) spectral output at 400 nm
Summary
A tunable light source with far-UVC output powered by a LDLS was presented and experimentally demonstrated. The in-band flux coupled by a 600µm core diameter UV fiber ranges from 329 µW and 1574 µW, the spectral density ranges from 284.5 µW/(nm*mm2) to 1124.6 µW/(nm*mm2), and the FWHM from 3.4 nm to 6.2 nm. Giving its superior properties of broad band coverage from 200 nm to visible range, high brightness, high-reliability light source and long life, the proposed TLS can be a good fit for certain applications, especially in phototherapy.
References
1. Richard A. Hobday, and John W. Cason, “The Open-Air Treatment of PANDEMIC INFLUENZA,” American Journal of Public Health, Supplement 2, Vol 99, No. S2, 2009.
2. David Welch, Manuela Buonanno, et al. “Far-UVC light: A new tool to control the spread of airborne-mediated microbial diseases,” Scientific Reports, 8: 2752 (2018)
3. Chukuka Samuel Enwemeka, Violet Vakunseh Bumah, et al. “Light as a potential treatment for pandemic coronavirus infections: A perspective”, J Photochem Photobiol B, 207: 111891, published online May 1, 2020
4. Donald K. Smith “Laser-driven light source,” US patent, Pub. No.: US7435982B2 (2006).
5. Donald K. Smith, “High brightness laser-driven light source,” US patent, Pub. No.: US9048000B2 (2015).